Augmented osteoplasty for proximal femur consolidation in cancer patients: biomechanical considerati
- F.H. Cornelis
- Dec 9, 2017
- 10 min read
Current advances in oncology have improved the mean overall survival of patients with metastatic bone disease [1]. Bone metastases are frequently localized at the trochanteric region and femoral neck, predisposing to pathological fractures [2]. These fractures may have serious consequences to the patients’ quality of life but also to their overall survival [3].
According to the literature, prophylactic consolidation is recommended in cases of lytic metastases of the proximal femur presenting a Mirels' score ≥8 [4–6]. While this weighted scoring system was proposed to quantify the risk of sustaining a pathologic fracture through any metastatic lesion in a long bone, the decision to perform surgical consolidation may also take into consideration the patient’s performance status and life expectancy. As surgery can have significant morbidity in cancer patients, minimally invasive treatment options may be considered in this fragile population [7].
Percutaneous osteoplasty has proved to be highly effective for the palliation of pain from bone metastases alone or in combination of other techniques such as ablation [8–11]. However, it was argued so far that it should be contraindicated for metastases that are located in the proximal femur because of inadequate bone consolidation during weight bearing [8,12–14]. Thus the rate of fractures despites osteoplasty is very high in the literature. In a retrospective analysis of 21 consecutives patients, fractures occurred in one third of the patients. And was significantly more frequent if a > 30 mm cortical rupture is associated [8,12–14]. In addition, revision surgery of proximal femur fracture that occurs despites osteoplasty is consider to be a challenging procedure [15]. To improve mechanical consolidation of osteoplasty, several authors have proposed to associate osteoplasty with the insertion of different type of devices in the femur [16–21]. These procedures are defined as augmented osteoplasties. The aim of this review was to detail the techniques of augmented osteoplasty proposed thus far in the literature and to report the early results in terms of safety and efficacy.
Biomechanical considerations for osteoplasty of the proximal femur
As bone cement (polymethyl methacrylate, PMMA) demonstrates biomechanical properties that are weak in tension but strong in compression, its benefit for vertebral body consolidation is appropriate. However, osteoplasty is ineffective in proximal femur because of the multiple stresses applied in this location during weight bearing [22,23] (Figure 1A). This is the reason why several authors believe that an intramedullary instrumentation is necessary for sufficient long-term consolidation of the proximal femur where rotational and shearing forces are applied [7]. The combination of cement and orthopedic devices has demonstrated a significant improvement in mechanical consolidation in the proximal femur [24]. Thus, the augmented osteoplasty may overcome therefore the limitations of osteoplasty alone reported so far.
Two concepts may be applied for augmented osteoplasty of the proximal femur as recommended in surgery [25]. Firstly, consolidation may only involve the femoral neck. Less invasive, this technique should be considered only for small lesion as consolidation covers only a limited area of the bone. Secondly, in case of larger lesion, it may be interesting to further lock the material using an intramedullary anchorage for instance. Although more complex to achieve, this concept allows better stabilization. However both require solid bone around the lesion as well as the use of cement for secure fixation of the devices.
Techniques of augmented osteoplasty.
Wires and cannulated screws
Percutaneous consolidation of impending pathological fracture of the proximal femur using osteoplasty and pins (such as Kirschner wire) or cannulated screws appeared feasible even under conscious sedation and imaging guidance [16,18,26]. Each devices (pin or screw) must enter a strong cortical bone, at best in three places following the "three point principle", to provide maximum resistance to rotation [27]. The devices inserted distally prevent varus angulation and the proximal devices prevent dorsal angulation of the femoral head [16]. However the configuration has to be adapted to the location and size of the tumor to ensure sufficient anchoring of the devices in healthy bone.
Tian et al. compared the efficacy of percutaneous osteoplasty with and without insertion of pins in 40 patients with Mirels’ score ranging from 8 to 12 [18]. The patients were placed in a lateral position on an operating table. After local anesthesia, a 13G bone puncture needle parallel to the axis of the femoral neck was inserted into the femur until the tip reached the bone cortex of the femoral head. A second needle was completely inserted parallel of the vertical axis of thighbone into the femur body. When consolidation was performed, a modified trocar needle was implanted into the proximal femur through the bone puncture needle sheath. A maximal amount of PMMA as possible (mean 31.2 ± 6.3 ml; 21-45) was injected into the femur through the bone puncture needle, including the lesion. The procedure time was 80 ± 7 minutes.
In 2012, Deschamps et al. reported a 100% technical success for osteoplasty and associated with the insertion of three cannulated screws in a study on 12 patients with metastasis of the proximal femur and mean Mirels’ score of 9.8 ± 1.2 (range, 8–11) [16] (Figure 2). The procedure was performed with 8 mm cannulated screws. The first screw was inserted along the inferior cortical of the femoral neck. The two others are placed against the upper cortical femoral neck—one posteriorly and the other anteriorly following an inverted triangle configuration. This configuration of screw placement was considered to be optimal for consolidation. Then PMMA was injected around the screws. The procedure was performed under general anesthesia (n = 6) or conscious sedation (n = 6). The mean duration was 110 ± 43 (range, 60–180) minutes and the hospitalization stay of 4 ± 1.6 days (2-7) in average. An extended cohort on 35 cases (Mirels' score ranged from 8 to 12, mean: 10.1), involving 8 patients from the previous study, was reported in 2015 [19] and showed similar results. The mean duration of procedures was 110min and the mean hospitalization stay of 2 days.
Recently, a study confirmed the advantage of augmented osteoplasty by evaluating the outcomes and local evolution of 36 treated lesions [28]. Augmented osteoplasty by screw insertion was performed in 6 impending fractures of the femoral neck under CT/fluoroscopy or cone-beam CT guidance with favorable outcomes. A technical success was observed in 91.6 % with a hospital stay of 3 days. An improvement was observed in 87.1 % of lesions at 1-month follow-up.
The Rebar concept
Another technique proposed by Kelekis et al, the REBAR concept, consists to perform augmented osteoplasty with a metallic mesh of stainless steel micro-needles [17]. While a limited anchorage in the healthy bone was obtained, this combination easy to perform seems to provide the necessary stability against the shearing forces applied in long bones during weight bearing. Coaxially a metallic mesh consisting of 25–50 medical grade stainless steel micro-needles (22 G, 2–6 cm length) was inserted in 9 patients. A direct access to the lesion of interest was obtained by bone access needles. The bone access needle was introduced through the greater trochanter, following the natural lines of the Haversian canal system. PMMA for osteoplasty was finally injected under fluoroscopic control. The mean procedure length was of 60minutes.
The Y-Strut® device
In a prospective study published by Cornelis et al, 10 patients with Mirels' score 8-11 undergone Y-STRUT® (Hyprevention®, Pessac, France) implantation [20,21]. Initially, this device was dedicatedly designed to enhance biomechanical structure of the femur and to prevent osteoporotic fracture [29]. It consists of two implants of 8mm of diameter made of a radiotransparent polyether-ether-ketone (PEEK) polymer material (Figure). Under general anesthesia and CBCT guidance, the two implants composing the device were inserted along guide wires using a dedicated instrumentation ensuring the adequate connection in situ between the two components. The perforation of the implants allows to control injection of the PMMA after insertion of the device. The mean volume of cement injected was 9.2 ml (range 3 to 15 ml). The cement aims to increase the surface contact between the bone and the device and to anchor the device in the proximal femur in the 3 dimensions. The mean duration of intervention was 97 ± 28 min (range: 60 - 155 min) and hospitalization was 2.3 days (range: 1-5 days).
More complex than any other technique aforementioned, the consolidation obtained with this device was studied through biomechanical tests in vitro, on human femurs, in order to simulate falls on anatomical pieces [29]. The study demonstrated the potential of Y- STRUT® to improve the biomechanical performance of the proximal femur. The loadings until failure showed that the insertion of the implant increased significantly (P=0.05) both fracture load (+18%) and energy to fracture (+32%) of the implanted femurs in comparison with the intra-individual controls. The instrumented femur resisted the implementation of the non-instrumented femur fracture load for 30 cycles and kept its performance at the end of the cyclic loading. This was consistent with previous biomechanical side-impact testing on pairs of femur using the same methodology [24].
Clinical outcomes
Safety and Efficiency of augmented osteoplasty
The physiology of pain reduction after augmented osteoplasty of metastatic lesions in the proximal femur is multifactorial, including stabilization of osseous micro- fractures or macro-fractures, destruction of nerve endings, cytotoxicity, and osteolysis treatment [17]. For Deschamps et al, the recovery was rapid and for symptomatic patients (n = 8), pain decreased from 6.5/10 on the visual analogic scale (VAS) (range, 2–9) before treatment to 1/10 (range, 0–3) 1 month after [16]. Two minors complications were observed. No fracture occurred after a median follow-up of 145 days (range, 12–608). Similar results were observed on the extended cohort (n=35) from the same team for a mean follow-up of 205 days (24-1633) [19]. Pain decreased significantly from 70 on VAS to 10 at 1 month in average. However 2 (5.7%) fractures occurred and required open surgery for stabilization. The first fracture occurred 3 weeks after the procedure in a patient who had Mirel score of 12 and cortical disruption was 50 mm long, out of the recommendations. The three screws entered in a smooth– surfaced cortical bone not allowing adequate stabilization. In the second patient, the fracture occurred 7 months after the procedure after fall injury. Tian et al demonstrated the impact of augmented osteoplasty in terms of bone consolidation [18]. Adding pins to osteoplasty resulted in a reduced risk of fracture compared to osteoplasty alone (p=0.05) but also in better pain relief (89 vs. 57 %, P = 0.034). [18]. The pain decreased more significantly after augmented osteoplasty during all the follow-up (8.84 ± 3.78months) (P=0.05). Likewise, Cazzato et al. did not reported secondary fractures after screw insertion in 6 impending fractures of the femoral neck [28].
Following the REBAR intervention, imaging assessed implant’s long-term stability for a follow-up range of 2–36 months [17]. No complication was observed and pain decreased also significantly.
After Y-STRUT® implantation, while a pain relief was observed only at 2 months, one patient was diagnosed at 6 months follow-up with an asymptomatic femoral neck fracture associated with a fracture of the implant attributed to tumour progression and non-optimal placement of the implant [20,21]. The average follow-up duration was 305 days (range 246 to 393 days).
Conclusion
Preliminary results from various studies evaluating the benefits of augmented osteoplasty of the proximal femur demonstrate their feasibilities, safeties and efficiencies to prevent pathological fracture. In cancer patients considered as not surgical candidates, these minimally invasive procedures appear as a promising alternative. These preliminary results justify to further evaluate these treatments on a larger population.
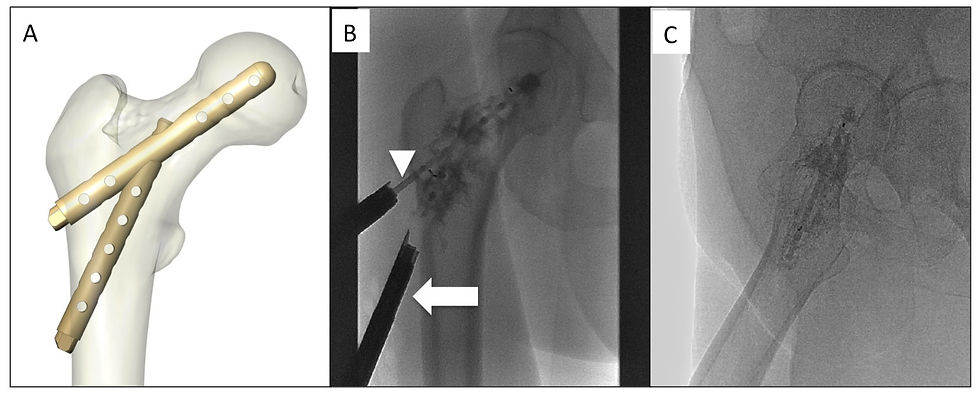
References
1. Nathan SS, Healey JH, Mellano D, Hoang B, Lewis I, Morris CD, et al. Survival in patients operated on for pathologic fracture: implications for end-of-life orthopedic care. J Clin Oncol. 2005;23:6072–82.
2. Schneiderbauer MM, Sierra RJ, Schleck C, Harmsen WS, Scully SP. Dislocation rate after hip hemiarthroplasty in patients with tumor-related conditions. J Bone Jt Surg. 2005;87:1810.
3. Parker MJ, Khan AZ, Rowlands TK. Survival after pathological fractures of the proximal femur. Hip Int. 21:526–30.
4. Mirels H. Metastatic disease in long bones. A proposed scoring system for diagnosing impending pathologic fractures. Clin Orthop Relat Res. 1989;256–64.
5. Jawad MU, Scully SP. In brief: classifications in brief: Mirels’ classification: metastatic disease in long bones and impending pathologic fracture. Clin Orthop Relat Res. 2010;468:2825–7.
6. Mavrovi E, Pialat J-B, Beji H, Kalenderian A-C, Vaz G, Richioud B. Percutaneous osteosynthesis and cementoplasty for stabilization of malignant pathologic fractures of the proximal femur. Diagn Interv Imaging. 2017;98:483–9.
7. Deschamps F, Farouil G, Hakime A, Barah A, Guiu B, Teriitehau C, et al. Cementoplasty of metastases of the proximal femur: is it a safe palliative option? J Vasc Interv Radiol. 2012;23:1311–6.
8. Anselmetti GC, Manca A, Ortega C, Grignani G, Debernardi F, Regge D. Treatment of extraspinal painful bone metastases with percutaneous cementoplasty: a prospective study of 50 patients. Cardiovasc Interv Radiol. 2008;31:1165–73.
9. Kelekis A, Lovblad KO, Mehdizade A, Somon T, Yilmaz H, Wetzel SG, et al. Pelvic osteoplasty in osteolytic metastases: technical approach under fluoroscopic guidance and early clinical results. J Vasc Interv Radiol. 2005;16:81–8.
10. Liu XW, Jin P, Liu K, Chen H, Li L, LI M, et al. Comparison of percutaneous long bone cementoplasty with or without embedding a cement-filled catheter for painful long bone metastases with impending fracture. Eur Radiol. 2016;27:120–7.
11. Wallace AN, Chang RO, Hsi AC, Jennings JW. Painful Pagetic vertebra palliated with percutaneous vertebral augmentation. Diagn Interv Imaging. 2016;97:269–72.
12. Dayer R, Peter R. Percutaneous cementoplasty complicating the treatment of a pathologic subtrochanteric fracture: a case report. Injury. 2008;39:801–4.
13. Chang SW, Murphy KPJ. Percutaneous CT-guided cementoplasty for stabilization of a femoral neck lesion. J Vasc Interv Radiol. 2005;16:889–90.
14. Basile A, Giuliano G, Scuderi V, Motta S, Crisafi R, Coppolino F, et al. Cementoplasty in the management of painful extraspinal bone metastases: our experience. Radiol Med. 2008;113:1018–28.
15. Beckmann J, Ferguson SJ, Gebauer M, Luering C, Gasser B, Heini P. Femoroplasty--augmentation of the proximal femur with a composite bone cement--feasibility, biomechanical properties and osteosynthesis potential. Med Eng Phys. 2007;29:755–64.
16. Deschamps F, Farouil G, Hakime A, Teriitehau C, Barah A, de Baere T. Percutaneous stabilization of impending pathological fracture of the proximal femur. Cardiovasc Interv Radiol. 2012;35:1428–32.
17. Kelekis A, Filippiadis D, Anselmetti G, Brountzos E, Mavrogenis A, Papagelopoulos P, et al. Percutaneous augmented peripheral osteoplasty in long bones of oncologic patients for pain reduction and prevention of impeding pathologic fracture: the rebar Concept. Cardiovasc Intervent Radiol. 2016;39:90–6.
18. Tian Q-H, He C-J, Wu C-G, Li Y-D, Gu Y-F, Wang T, et al. Comparison of percutaneous cementoplasty with and without interventional internal fixation for impending malignant pathological fracture of the proximal femur. Cardiovasc Intervent Radiol. 2016;39:81–9.
19. Deschamps F, de Baere T, Hakime A, Pearson E, Farouil G, Teriitehau C, et al. Percutaneous osteosynthesis in the pelvis in cancer patients. Eur Radiol. 2016;26:1631–9.
20. Cornelis FH, Tselikas L, Carteret T, Lapuyade B, De Baere T, Le Huec JC, et al. Percutaneous internal fixation with Y-STRUT® device to prevent both osteoporotic and pathological hip fractures: a prospective pilot study. J Orthop Surg Res. 2017;12:27.
21. Cornelis FH, Tselikas L, Carteret T, Lapuyade B, De Baere T, Cabane V, et al. A novel implant for the prophylactic treatment of impending pathological fractures of the proximal femur: results from a prospective, first-in-man study. Cardiovasc Intervent Radiol. 2017;40:1070–6.
22. Saha S, Pal S. Mechanical properties of bone cement: a review. J Biomed Mater Res. 1984;18:435–62.
23. Lewis G. Properties of acrylic bone cement: state of the art review. J Biomed Mater Res. 1997;38:155–82.
24. Beckmann J, Springorum R, Vettorazzi E, Bachmeier S, Lüring C, Tingart M, et al. Fracture prevention by femoroplasty-cement augmentation of the proximal femur. J Orthop Res. 2011;29:1753–8.
25. Haidukewych GJ. Metastatic disease around the hip: maintaining quality of life. J Bone Jt Surg - Br Vol. 2012;94–B:22–5.
26. Abdel-Aal AK, Underwood ES, Saddekni S. Use of cryoablation and osteoplasty reinforced with Kirschner wires in the treatment of femoral metastasis. Cardiovasc Intervent Radiol. 2012;35:1211–5.
27. Bout CA, Cannegieter DM, Juttmann JW, Willenegger H. Percutaneous cannulated screw fixation of femoral neck fractures: the three point principle. Injury. Springer Verlag, Heidelberg; 1997;28:135–9.
28. Cazzato RL, Koch G, Buy X, Ramamurthy N, Tsoumakidou G, Caudrelier J, et al. Percutaneous image-guided screw fixation of bone lesions in cancer patients: double-centre analysis of outcomes including local evolution of the treated focus. Cardiovasc Intervent Radiol. 2016;39:1455–63.
29. Szpalski M, Gunzburg R, Aebi M, Delimoge C, Graf N, Eberle S, et al. A new approach to prevent contralateral hip fracture: Evaluation of the effectiveness of a fracture preventing implant. Clin Biomech. 2015;30:713–9.
Comments